Introduction
This section is about our simulation of what is observed at CERN within a detector,
showing you what to look for and how a new particle might be identified, in this case, the Higgs Boson.
Accelerators boost particles to high energies, around ~7 TeV at CERN,
so that when they collide, there is ~14 TeV of energy giving ample energy to create a Higgs boson.
The Higgs boson has a mass of (125.18 ± 0.16) GeV which means that, when it decays, all of the lighter decay
products have significant energy. This energy corresponds to a significant momentum due to the relatively low masses of the decay products, e.g a b-quark with 5 GeV.
To measure the momentum, electromagnets are placed around the detectors which generate strong magnetic fields. These magnetic fields curve the path of the particles depending on how much momentum they have. Lower momentum particles will spiral around the inner detector whereas high momentum particles will travel in a straight line. This effect can be seen within the inside ring of the simulation.
How is a detector laid out?
Within each detector, there are several 'layers' called calorimeters, where each calorimeter measures the energy of different particles. Calorimeters work by forcing the particles to stop entirely or absorb the particles from a collision, thus depositing their energy within the detector. Each calorimeter is displayed by a different ring.
The innermost ring represents the Electromagnetic Calorimeter (ECAL) which detects electrons and photons as they interact with electrically charged matter.
The next ring going outwards represents the Hadron Calorimeter (HCAL), aptly named as it measures hadronic energy. A hadron is a particle that is made of more than one quark, so all baryons (made of three quarks, i.e. protons and neutrons) and mesons (made of two quarks, i.e kaons and pions). But you have to be careful with some particles such as pions, as they will leave some energy in the ECAL too thus creating two energy towers, one in each calorimeter.
The third ring represents the muon detector, which counts the number of muons passing through it and attempts to measure the energy of them. Muons are highly energetic and travel at very high velocities, which when combined with their low interaction rate, makes them very penetrative and so the muon detector is around the outside of the detector. This means that the muons have a higher chance to interact having travelled through the detector beforehand.
A Higgs boson being detected by the ATLAS detector
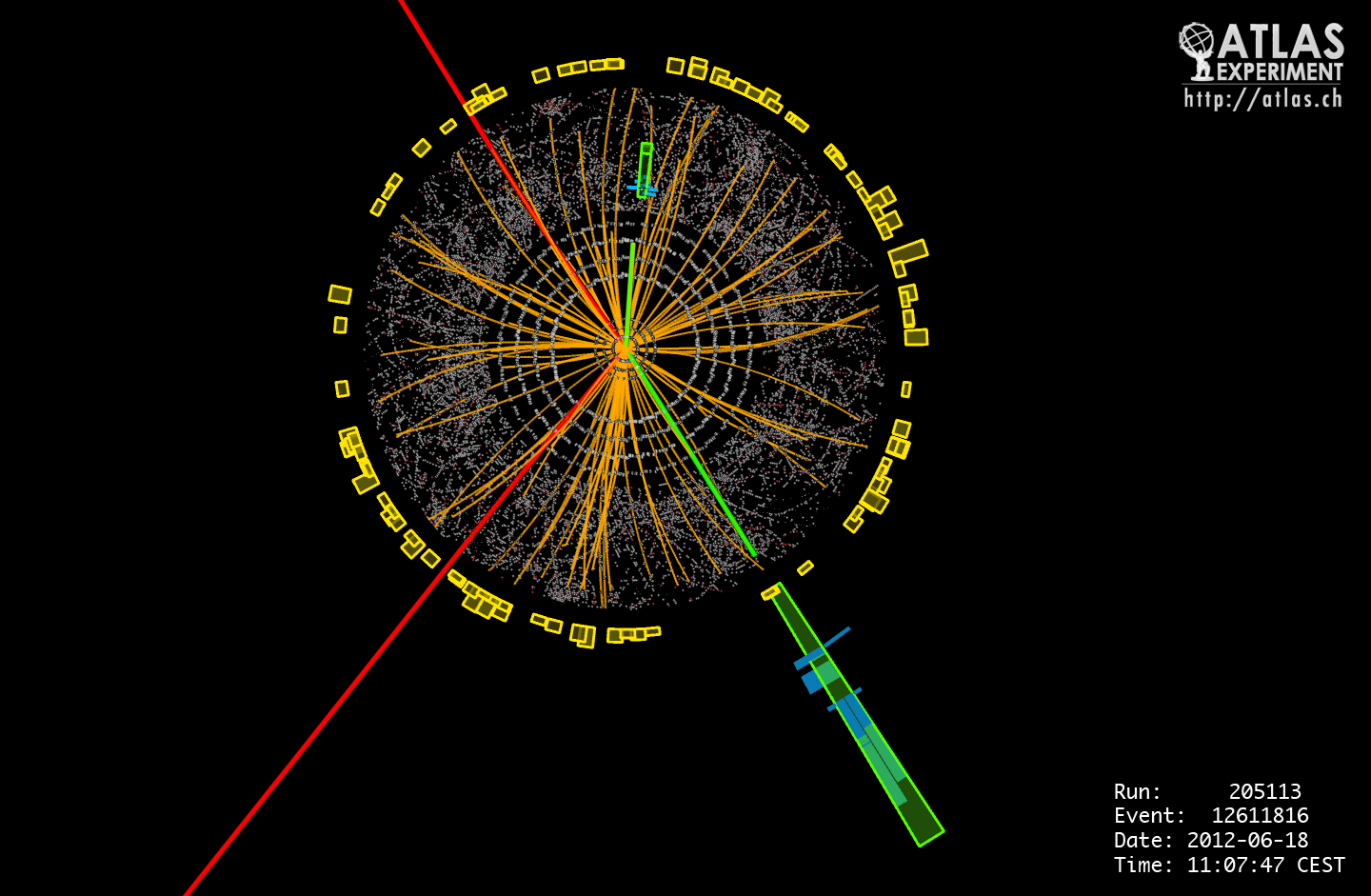
What to look for?
The Higgs Boson has four main decay channels:
- H → γγ (Higgs boson to two photons 0.0229 %)
- H → ZZ → llll (Higgs boson to two Z bosons to four leptons 0.0131 %)
- H → bb̅ (Higgs boson to a b-quark and anti-b-quark 56.9 %)
- H → ττ (Higgs to a tau lepton and an anti-tau lepton 6.28 %)
The branching ratios above indicate how unlikely it is for these decay modes to occur, let alone detected after creating a Higgs boson. To counter this, thousands of runs are done to achieve a high precision to the branching ratios. To achieve this, the collaboration of 22 member states started research in the LHC on 30 March 2010, to announce a new particle on the 4th July 2012 with further measurements being taken till February 2013 (the start of ).