Hypothesis
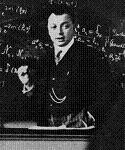
Wolfgang Pauli
Wolfgang Pauli can be seen as the somewhat reluctant father of the neutrino, being the first one to predict its existence but not being very happy to do so! Researchers had noticed that nuclear beta decay seemed to break the laws of conservation of energy and linear and angular momentum. The energy of the beta radiation followed a continuous spectrum. This was surprising since other types of radioactive decay known at that time (alpha decays) emitted radiation with discrete energy levels.
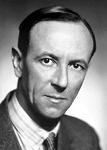
James Chadwick
Pauli proceeded to propose a "desperate remedy" to explain that puzzling situation and to save the conservation laws. Unable to attend a physics meeting in December 1930, he sent a letter to the other "radioactive ladies and gentlemen" presenting what his simple but unheard-of way of thinking had led him to propose. He suggested that the missing energy was being carried away by a third particle emitted in the decay. It seemed that Pauli himself was not very comfortable with it. In the first place, it took him some time to go public with his idea at all, and even then he apologized for predicting a particle that he thought would be impossible to detect since it had no electric charge and therefore was not very likely to interact with matter. Also, the particle was believed to have virtually zero mass and be spin half. Pauli called that particle the neutron and it would be emitted together with the electron in beta decay so that the total energy and momentum would be conserved.
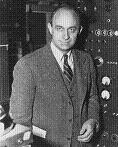
Enrico Fermi
Two years later, James Chadwick discovered what we now call the neutron, but it was evident that this particle was far too massive to be the neutron Pauli had predicted. However, his elusive particle played an important role in the first theory of nuclear beta decay formulated by Enrico Fermi in 1933. Since the name neutron was now taken, Fermi gave it the name neutrino by which it goes today, meaning "the little neutral one". Despite Fermi's work in the 1930s, the neutrino at first did not draw many physicists' attention; in fact, it was considered to be a somewhat "arrogant" particle, which simply ignored matter and whose one and only task was to carry off energy in beta decay.
On 16 January 1934, Enrico Fermi submitted for publication his famous paper on the first viable theory of the weak force, which is responsible for beta decay. He explained the beta decay of a nucleus in terms of the creation and immediate emission of an electron simultaneously with an invisible neutrino. However, his paper was rejected by the journal Nature, because "it contained speculations too remote from reality to be of interest to the reader".
Discovery
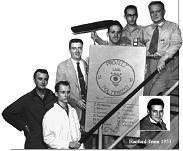
Hanford neutrino team
Pauli himself was so thoroughly convinced that the neutrino could not be detected that he actually bet a case of champagne that nobody would ever detect the neutrino. Luckily, he was proven wrong when the first Nobel-Prize winning detection of neutrinos in 1956 by Fred Reines and Clyde Cowan of Los Alamos cost him a case of champagne. After all, he seemed quite pleased with himself, sending back a telegram reading: "Thanks for the message. Everything comes to him who knows how to wait."
Standard Model
The standard model provides a powerful picture of particle physics altogether and can be regarded as the basic theory of all matter. It is based on three "generations" of fundamental spin 1/2 particles called quarks and leptons. The neutrino is a lepton and comes in three different "flavours": the electron neutrino in the first generation, the muon neutrino in the second generation and the tau neutrino in the third generation. In the standard model, they are massless and there is no known generation-mixing flavour-changing mechanism provided in the leptonic sector. In the quark sector, however, cross-generational flavour-mixing is included and was observed in detectors at the point of interaction. Fundamental particles interact with each other by exchanging gauge bosons with spin 1, which are also called force carriers. Photons mediate the electromagnetic force, the W+, W- and Z0 particles carry the weak force and eight gluons carry the strong force. Quarks interact via all three of these forces, whereas charged leptons interact via the electromagnetic and the weak force, and neutral leptons interact only via the weak force. The force of gravity is not included in the model, because at the energies and masses relevant to the standard model it is insignificant. The standard model provides a theory of fundamental constituents with which one can describe the structure and behaviour of all forms of matter.